Muon diagnostics is a new technique of remote monitoring and forecasting of dynamic processes in the heliosphere and in the magnetosphere and atmosphere of the Earth based on the analysis of spatial-angular and temporal variations of ground-level muon flux simultaneously detected from all directions of the upper hemisphere in hodoscopic mode.
The intensity of cosmic rays (CR) at ground level (mainly muons) varies under the impact of atmospheric conditions. Besides of these phenomena of a local character, there are global reasons of cosmic ray intensity modulations determined by geophysical cyclic processes and processes related with solar activity. Thus, muon flux variations bring information about both atmospheric and extra-atmospheric processes, and their separate investigation demands the development of new experimental approaches and new data analysis techniques. Basic conceptions of the theory of such CR variations of atmospheric and extra-atmospheric origin were developed in the middle of XX century [1]. Now, with the creation of a new generation of ground level muon detectors—wide-aperture muon hodoscopes with high angular resolution—the possibility to study dynamic processes in the Earth's atmosphere and near-terrestrial space is opened.
PHYSICAL PRINCIPLES OF MUON DIAGNOSTICS
Cosmic ray muon measurements allow conduct real-time investigations of spatial-angular dynamics of both primary and secondary cosmic ray fluxes. Such approach is based on the fact that secondary muons keep (with taking into account the Earth magnetic field and interplanetary magnetic field) the direction of primary particles motion. Thus, the measurements of muon flux in hodoscopic mode give a chance to study the structure of various perturbations in the heliosphere.
During the powerful solar coronal mass ejections (CME), clouds of magnetized plasma can produce strong magnetic storms in magnetosphere of the Earth. When solar plasma cloud reaches the Earth vicinity, decreases of CR flux (Forbush effects) registered by worldwide net on neutron monitors (NM) and muon detectors take place. Movement of solar plasma through the heliosphere disturbs magnetic field causing the deflection of galactic CR (see Fig. 1) and hence of muons generated in upper layers of the atmosphere and directed at plasma clouds. Thus, spatial-angular variations of muon flux can be used for localization of heliosphere disturbances and as a precursor of perturbation of the Earth’s magnetosphere.
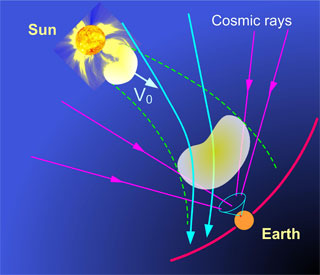
Fig. 1. Deflection of galactic cosmic rays related with solar activity.
On the other hand, muon flux at the ground level is strongly related with different thermodynamic processes in the Earth’ atmosphere at generation level (barometric, temperature effects) and with more complex wave processes in low stratosphere (inner gravitational waves of air density, significant density gradients, etc.), correlated with different turbulent and wave processes of geophysical origin (powerful thunderstorms, hurricanes, tornados, etc.), which are localized in space and time (see Fig.2). Wave processes at the altitude of muon generation modulate muon flux and can be used as precursor of origin and development of such phenomena at large distances from the muon detector.
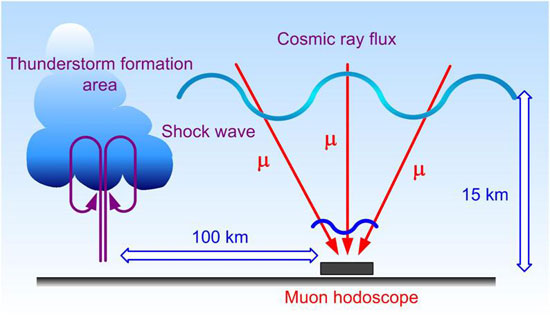
Fig. 2. Modulations of cosmic ray muon flux in upper layers of the atmosphere.
The registration of spatial-angular dynamics of muon flux gives unique initial data for the solution of inverse task – the study of cosmic ray modulation processes using the analysis of spatial and temporal variations of muon flux at the Earth’s surface.
DETECTOR REQUIREMENTS
For the first time, cosmic ray variations during strong magnetic perturbations (Forbush decreases) were detected in muon flux [2]. The further study of cosmic ray variations at the Earth’s surface was conducted mainly by means of neutron monitors, which can measure only integral flux. Traditional muon telescopes can detect flux of atmospheric muons from one or several directions but do not have sufficient aperture and angular resolution to study spatial and temporal dynamics of muon flux.
In order to use the principles of muon diagnostics, the wide-aperture muon detectors—hodoscopes, which give the possibility to simultaneously measure the intensity of muons from all directions of upper sky hemisphere, are necessary. Such detectors must have high angular resolution of muon track reconstruction (of the order of 1 degree) and large sensitive area to provide necessary statistical accuracy of experimental data for all zenith and azimuth angular bins (on the ground level, of the order of 106 events per hour). As total intensity of muon flux at the Earth’s surface is about 0.01 cm –2 s -1, the area of detector must be about 10 m2 or larger. At present, two muon hodoscopes are under operation in Moscow Engineering Physics Institute: TEMP [3] and URAGAN [4].
The first in the world muon hodoscope TEMP operates since 1996. TEMP (see Fig. 3) consists of two pairs of horizontal coordinate planes (X, Y) with sensitive area of 9 m2. These pairs are vertically separated by 1 m distance. Each plane is assembled of narrow scintillator counters (2.5 cm x 1 cm x 300 cm) viewed by PMT. Total number of counters is 512; angular resolution 1 – 2°. Data are continuously registered as intensity arrays with dimension 255 x 255 directional cells. TEMP is located in the basement of the building; threshold muon energy is about 0.5 GeV.
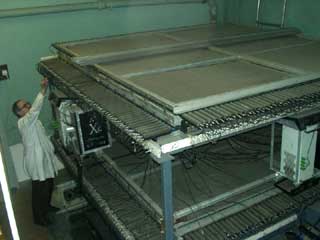
Fig. 3. Muon hodoscope TEMP.
In 1998–2001, the Russian-Italian coordinate detector DECOR [5] was deployed around the Cherenkov calorimeter NEVOD [6]. Top supermodules of DECOR are located on the cover of the calorimeter water pool and are assembled of eight horizontal streamer tube chamber layers. In 2005, on the basis of top DECOR supermodules a new multipurpose muon hodoscope URAGAN (Fig. 4) was constructed.
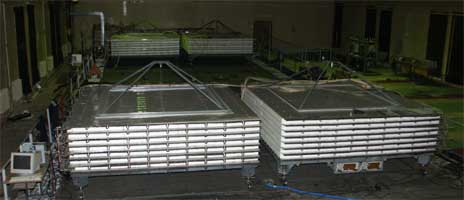
Fig. 4. Two supermodules of muon hodoscope URAGAN.
The URAGAN supermodule includes eight planes interlaid with 5 cm foam plastic and composed of 320 streamer tubes (1 cm x 1 cm x 350 cm) with external strips (along and across streamer tubes) forming two-dimensional readout system. Total area of each SM is about 11.5 m2 and it includes 4864 data channels. The data processing system allows reconstruct muon tracks in the on-line mode and to register muon flux from the upper hemisphere as continuous sequence of 2D-pictures (Fig. 5). The setup provides detection of particles in a wide range of zenith angles (from 0 to 80°) with angular accuracy about 0.7°. Threshold muon energy is 0.2–0.6 GeV (for different zenith angles).
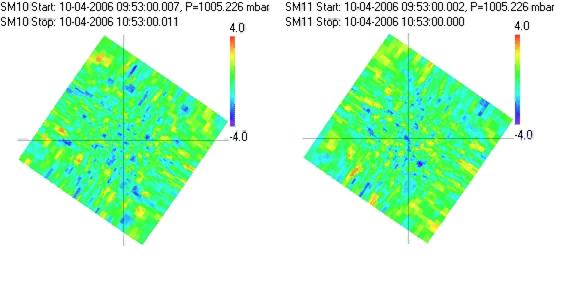
Fig. 5. A "muon snap-shots" (in standard deviations from the average) of upper hemisphere made with two supermodules of URAGAN hodoscope.
Now three supermodules of URAGAN with total area 34.5 sq. m are under operation in MEPhI (Moscow). The total muon rate is about 250 thousand muons per minute, which provides statistical accuracy about 0.2 %.
First results [7–9] of hodoscopes' data analysis demonstrate a rich forecasting potential of muon diagnostics since such approach allows detect disturbed domains and observe the dynamics of their development in real-time regime. In Fig.6, the preliminary URAGAN data on anisotropy of muon intensity during the Forbush decrease of May 15, 2005 are shown.
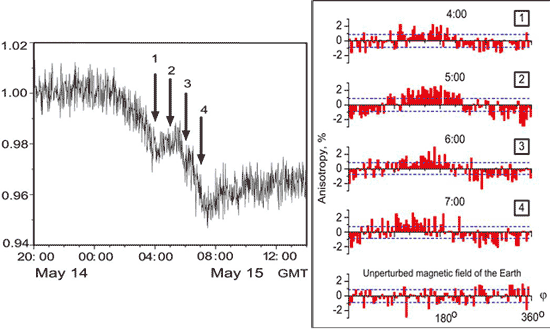
Fig. 6. Total normalized muon counting rate of URAGAN supermodule during Forbush decrease (left) and the dynamics of relative azimuth anisotropy of muon flux (right).
In the right panel relative deviation of muon flux from the average one as a function of azimuth angle is plotted for different time moments during Forbush decrease 15 May 2005 (left panel): 1 – 4:00, 2 – 5:00, 3 – 6:00, 4 – 7:00 (UTC). The results have been obtained by summation of muon numbers from 25° to 65° zenith angles. Coordinate system is attached to the NEVOD calorimeter with X-axis directed to South-West. From the figure, a steady gradual shift of muon flux asymmetry direction by approximately 60 degrees (from 180° to 120°) is seen. Such variations can be explained by the Earth rotation (during three hours) relative to solar plasma cloud coming to the Earth.
2D-variations of muon rate are sensitive to active processes in the Earth's atmosphere and can be used for early recognition of local powerful hurricanes, tornados etc. Modern data analysis techniques are used for searching of probable predictor signals of such phenomena. For example, during 26 June, a strong hurricane generated by powerful turbulent processes at the atmospheric front appeared in Dubna town in the North of Moscow region at 12:00 of local time. Dubna is located about 140 km far from Moscow. In Fig. 7, existence of two waves with periods about 95 and 70 minutes was found. These waves appeared several hours before the moment of hurricane arrival in Dubna, indicated in the picture by a gray strip.
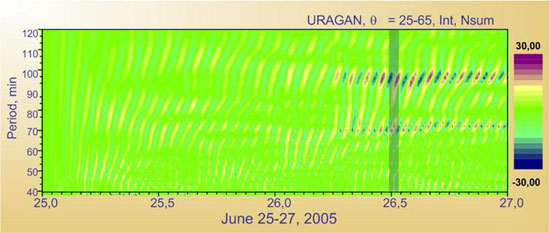
Fig. 7. Wavelet transformation of URAGAN muon rate during June 25–27, 2005.
CONCLUSION
The use of cosmic rays, in particular muons, as a penetrating component and muon hodoscopes as apparatus for detection of “muon images” of atmosphere and extra-terrestrial space opens a new perspectives for remote monitoring of environment. Registration of muon flux during heliosphere perturbations (like CME, magnetic clouds, solar energetic particles, and so on) using even a single wide-aperture hodoscope gives a possibility to obtain a unique information about the structure and dynamics of such events and to compare the predictions of various models of heliospheric processes with direct measurements of muon flux variations. The use in future of the world-wide net of muon hodoscopes may substantially widen the possibilities of ground-based space weather monitoring. The use of muon hodoscopes for diagnostics of active phenomena in the Earth's atmosphere will give a possibility to watch the inner atmospheric processes at principally new level.
In frame of International Heliophysical Year, a special Coordinated Investigation Program "Muon diagnostics of heliosphere and the near-Earth space" (CIP58) has been opened. This project is directed to developing of muon diagnostics ideas including international cooperation.
REFERENCES
- L. I. Dorman, Cosmic Rays in the Earth's Atmosphere and Underground, 2004 Springer-Verlag and references therein.
- S. E. Forbush, On the Effects in the Cosmic-Ray Intensity Observed During Magnetic Storms, Phys. Rev., 1937, V. 51, p. 1108.
- V. V. Borog et al., Large aperture muon hodoscope for studies in solar-terrestrial physics, Proc. 24th ICRC, Rome, 1995, V.4. p. 1291.
- D. V.Chernov et al., Experimental setup for muon diagnostics of the Earth’s atmosphere and magnetosphere (the URAGAN project), Proc. 29th ICRC, Pune, 2005. V.2. p. 457.
- M. B. Amelchakov et al., Proc. 27th ICRC, Hamburg, 2001, V. 3, p. 1267.
- V. M. Aynutdinov et al., Astrophysics and Space Science, 1998, V. 258, p. 105.
- N.S. Barbashina et al., Proc. 29th ICRC, Puna, 2005, V. 2, p. 377.
- I.I. Yashin et al., Proc. 20th ECRS, Lisboa, 2006.
- N.S. Barbashina et al., astro-ph/0701288.
|